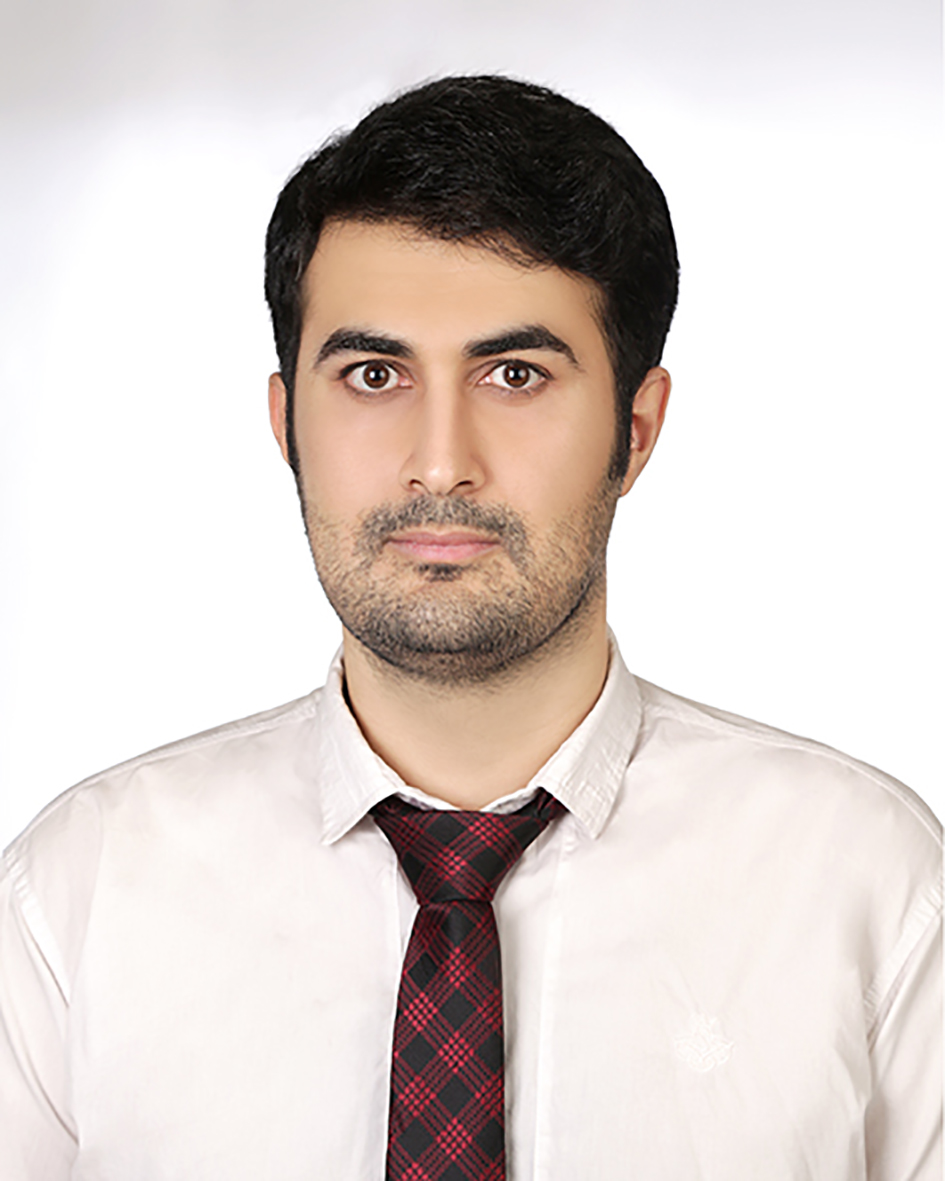
About me
I am a Mechanical Engineer with a Master's degree from the University of Toronto. My expertise lies in the numerical simulation of particulate flows and complex fluid flows within microfluidic devices. In addition to theoretical knowledge, I design and fabricate microfluidic devices, including cell and microparticle separators as well as Organ-on-a-chip devices. I am passionate about using artificial intelligence to address real-world problems.
My skills
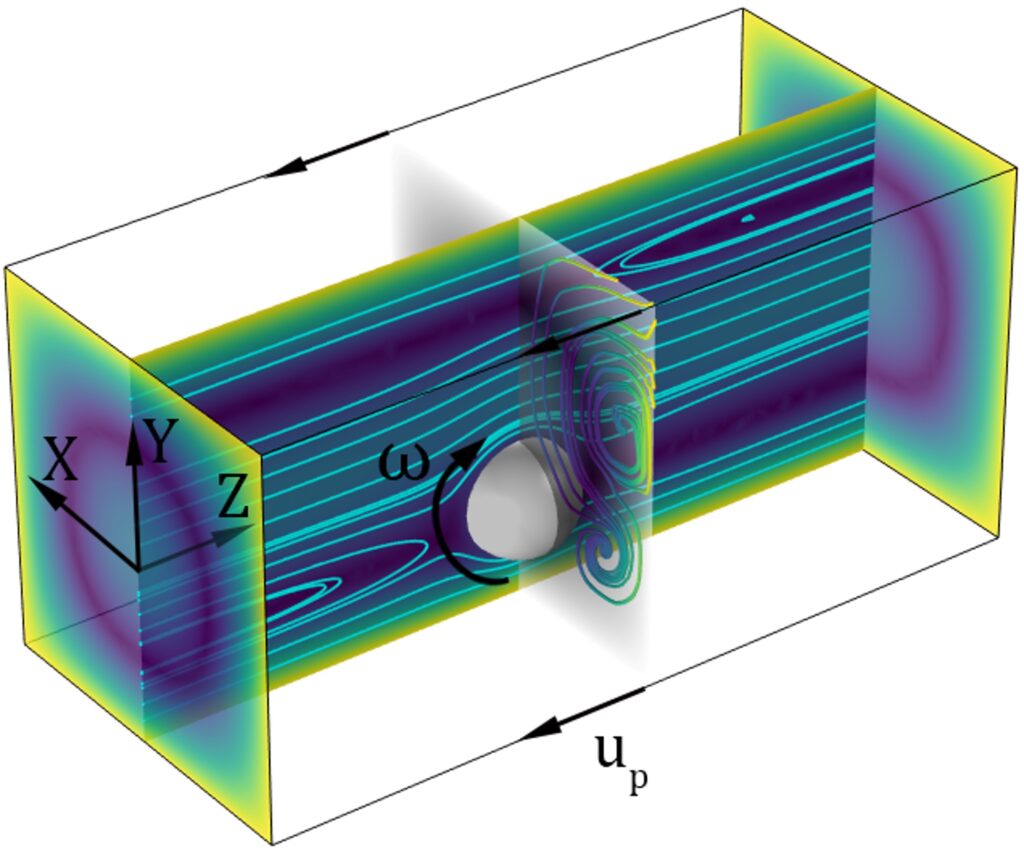
Numerical Simulations
I use common modeling and analysis packages, such as COMSOL Multiphysics, to simulate complex fluid flows in microfluidic devices. I developed a direct numerical simulation (DNS) model to increase the accuracy of simulations.
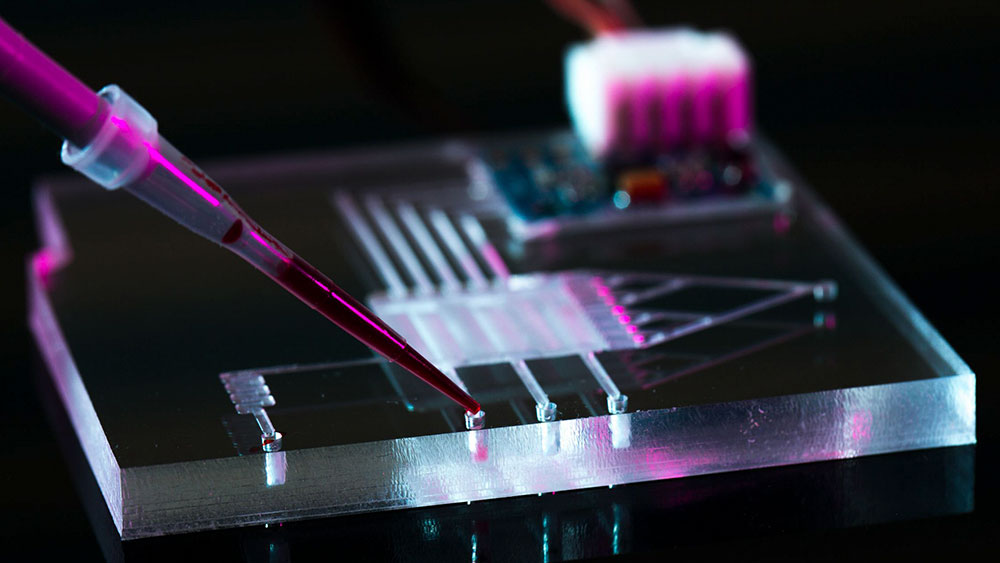
Soft lithography
I have experience in microfabrication using the prevalent soft lithography method to create microfluidic devices. In this method, first, a master mold is fabricated using SU-8 photoresist and a photomask. Then, PDMS is then poured, cured, and peeled off from the mold. The PDMS is finally sealed to a glass slide with a plasma binder.
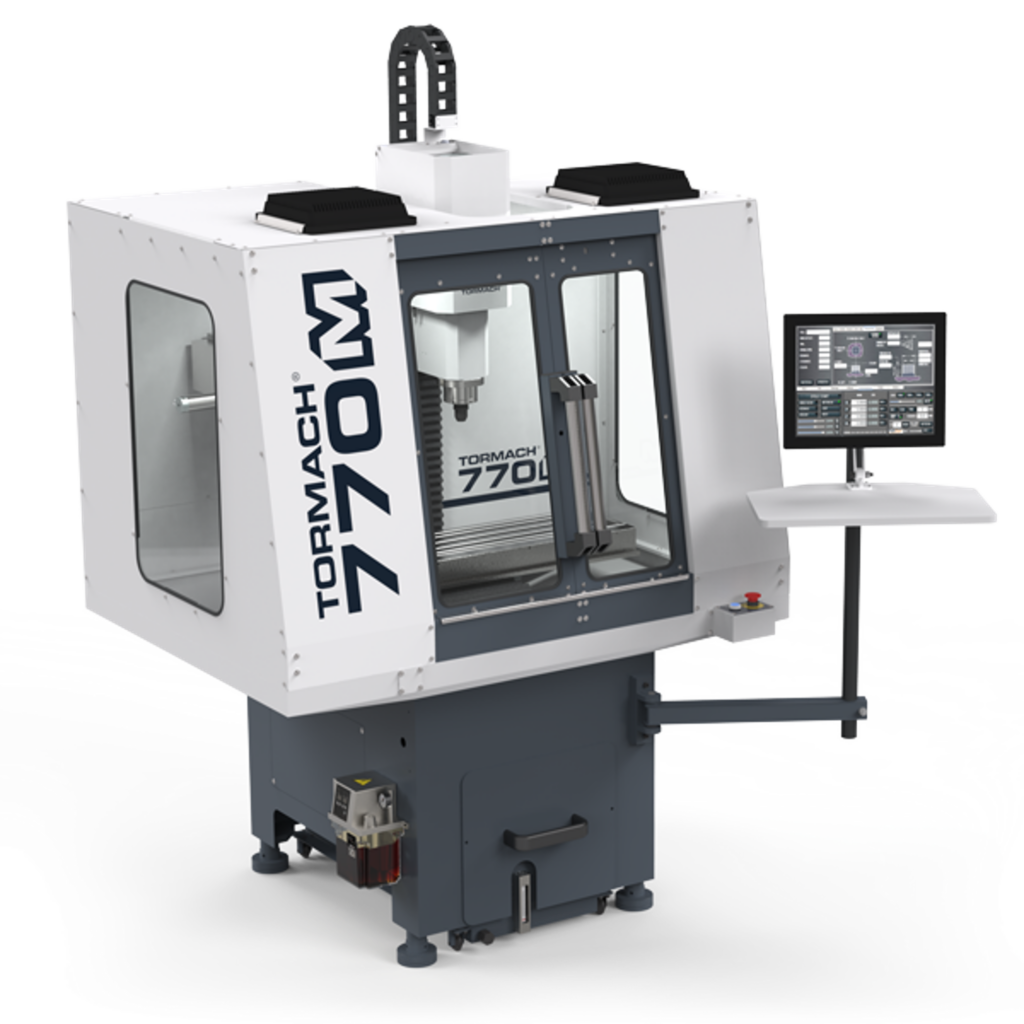
CNC Machining
I utilized CNC micro-milling to fabricate microfluidic devices. In this approach, first, a G-code is generated based on the 3D CAD design, drawn in Fusion 360 or SolidWorks. This code guides the CNC machine to carve the design onto a PMMA sheet. The carved layer is then thermally bonded to a raw PMMA layer to seal the microfluidic channels.
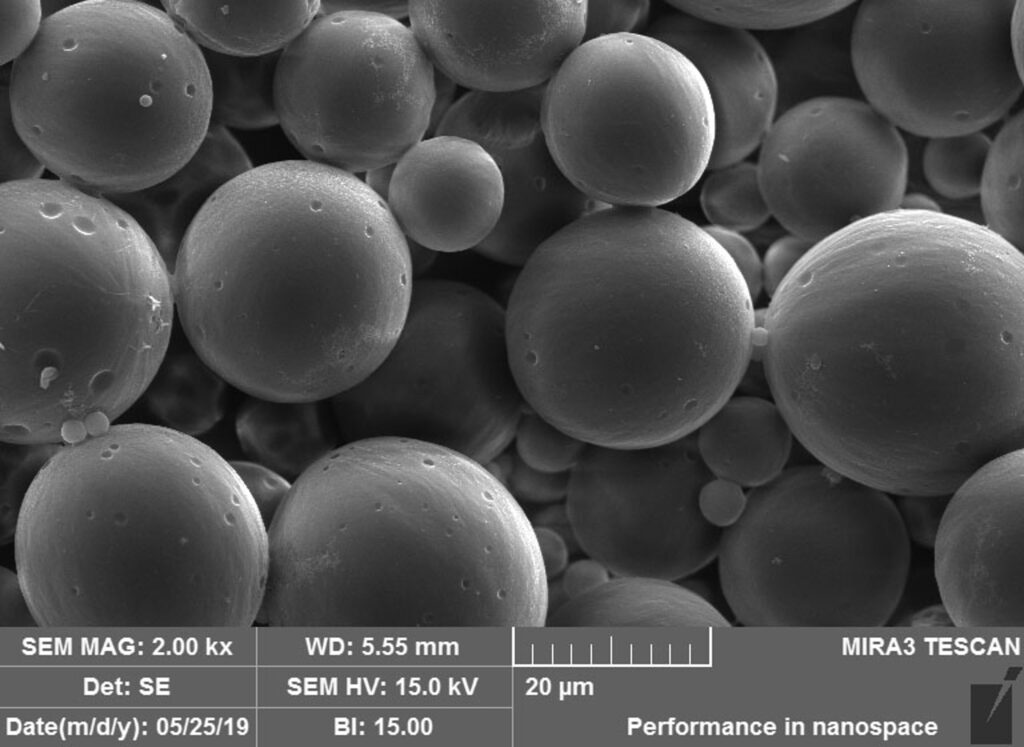
Microsphere Fabrication
I have experience in fabricating microspheres using the conventional double emulsion method, specifically for water-in-oil-in-water (W/O/W) emulsions, which are used for encapsulating drugs and active ingredients.
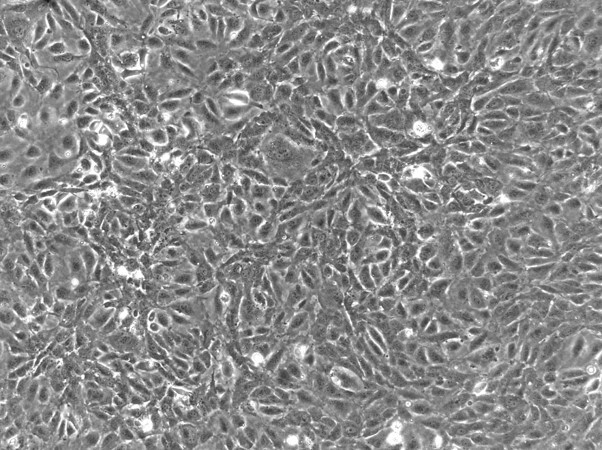
Cell Culture
I have experience in cell culture techniques and have conducted cell viability assays on a few cells, including the MCF-7, MDA-MB-231 breast cancer lines, and Human Umbilical Vein Endothelial Cells (HUVEC).
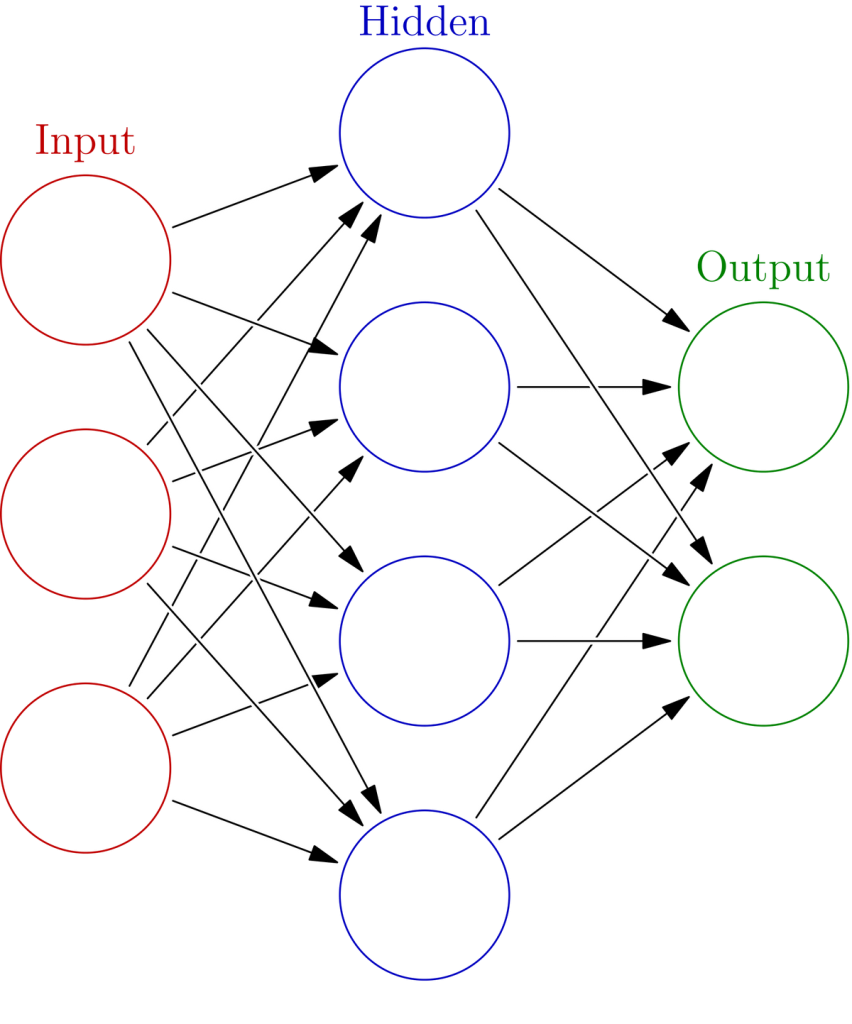
Machine Learning
This is the area that I have recently started to explore through developing a Deep Learning-CFD hybrid model for simulation of particulate flows in microfluidics devices.
Project
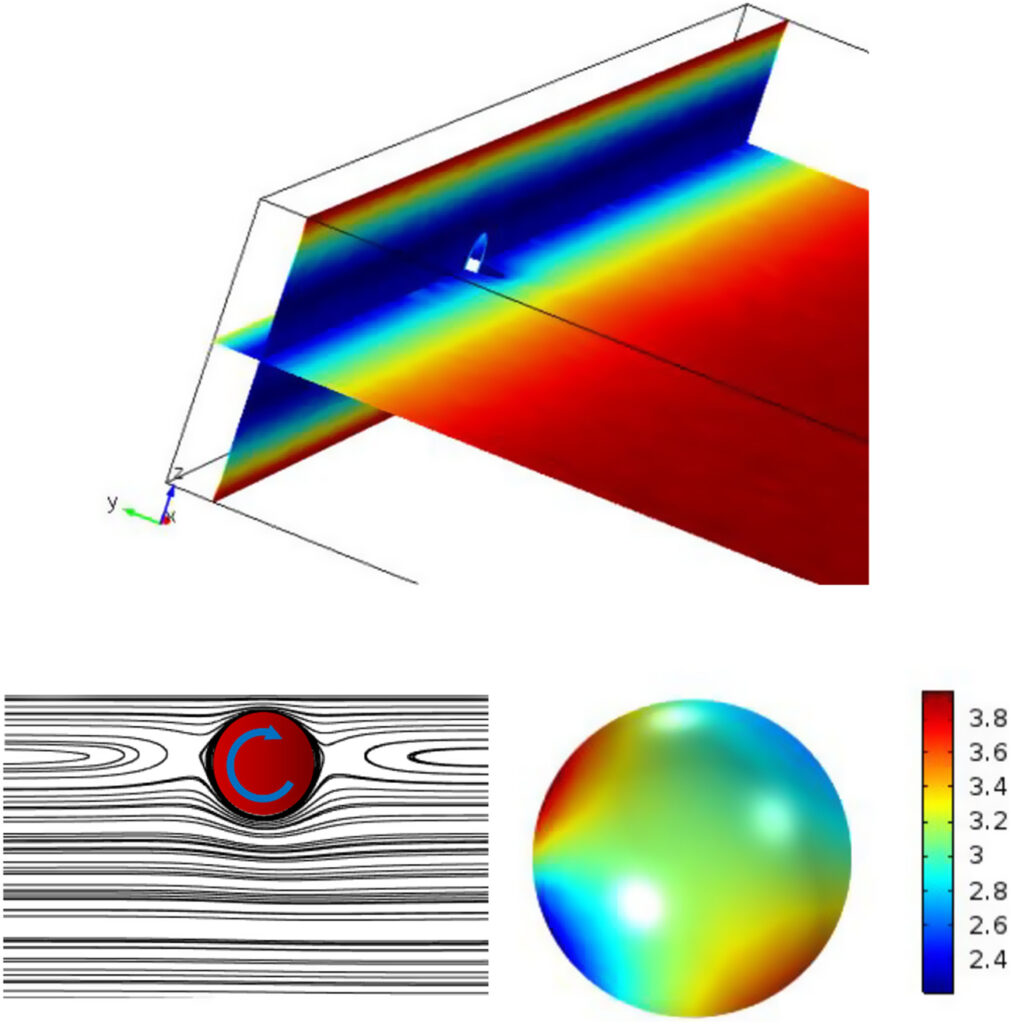
Inertial particle focusing in serpentine channels on a centrifugal platform
This project utilizes a centrifugal platform to move Newtonian fluid and particles in serpentine channels in order to focus particles at the outlet of the channel. Direct Numerical Simulation (DNS) is performed to calculate the inertial lift force acting on particles in the cross section of the channel. The effects of different parameters, including the corner angle of the channel, particle size, and particle density, on the focusing performance of the serpentine channel are investigated.
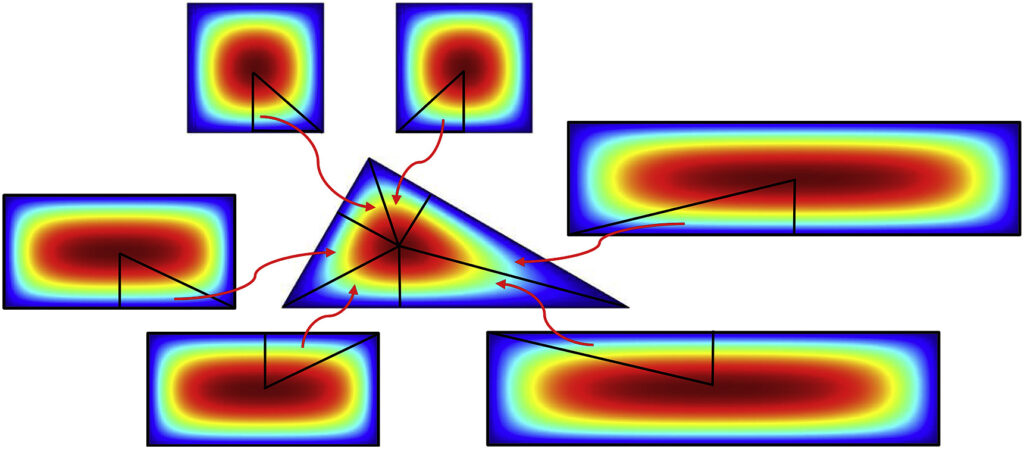
A method for fast prediction of inertial focusing patterns in non-rectangular channel cross-sections
In this project, inertial particle focusing in rectangular channels has been investigated. Based on these findings, a analytical method has been proposed to predict the focusing patterns of particles in channels with non-rectangular cross-sections. This method is based on the idea that the velocity profile of a non-rectangular cross-section can be represented by a superposition of velocity profiles from rectangular channels. As the inertial lift force is correlated with the velocity profile, it is hypothesized that particle focusing in the non-rectangular section is analogous to that in rectangular channels.
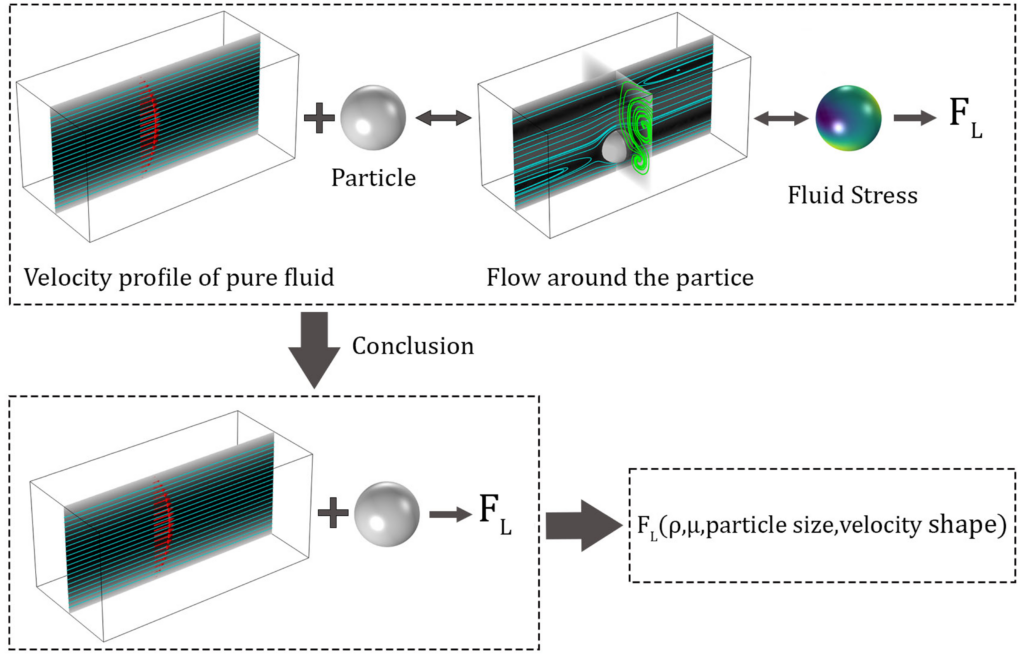
Developing a Deep learning-CFD hybrid model for predicting cells and micro-particles trajectories in the microfluidic devices
Experimental optimization of microfluidics cells and particles manipulators is often inefficient and time-consuming, while Direct Numerical Simulation (DNS) is computationally expensive. To address these challenges, I propose a novel geometry-free variable set to reformulate the conventional inertial lift force formula. A deep learning neural network has been trained on force data collected across various microchannel shapes to decipher the relationship between the lift force and these variables. This model can quickly predict the trajectories of particles in various microfluidics devices.
- Manuscript Under Preparation
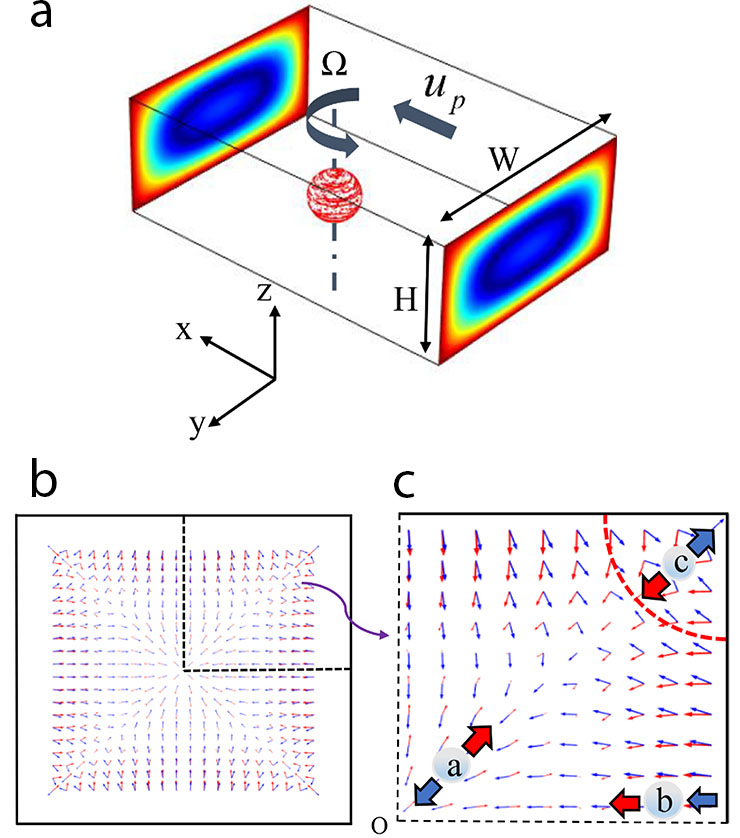
Particle motions in viscoelastic fluid flows
Particle motions in viscoelastic fluid flows In this project, the focusing patterns of particles in the cross-sections of straight channels with square and rectangular geometries are examined, considering various flow rates and concentrations of PEO solution, a viscoelastic fluid. Figure a illustrates the modeling of particle motion in a segment of a straight channel, with fluid flow directed in the x-axis. The flow of the polymeric fluid is modeled using the Oldroyd-B constitutive equation. Figure c shows vector field plot of inertial lift (red vectors) and elastic (blue vectors) forces in the first quarter of cross section of the square channel for three significant areas (locations of particles a, b and c). Due to symmetry, only the first quarter is considered.
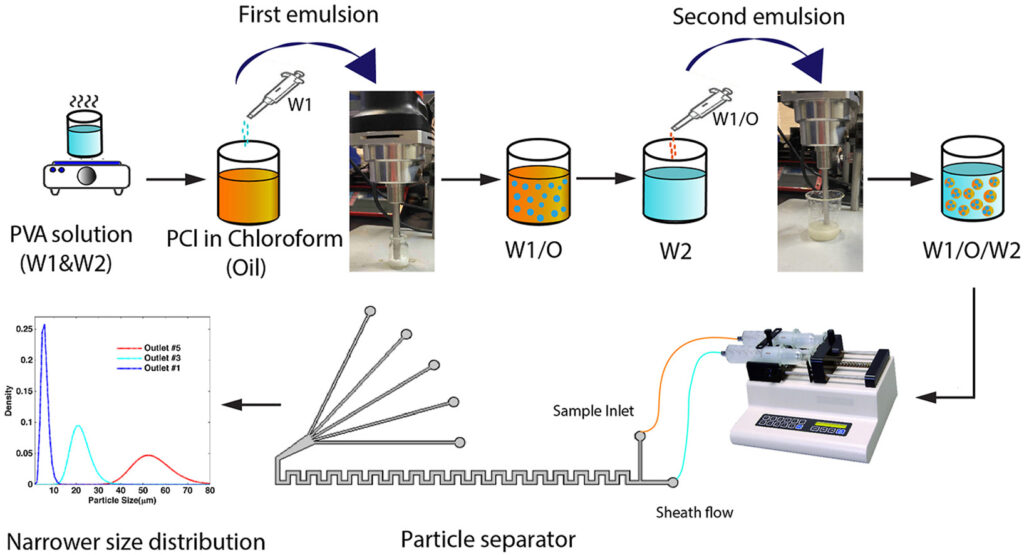
An integrative method to increase the reliability of conventional double emulsion method
In this project, the effect of different parameters of the conventional double emulsion method on microsphere morphology and size has been investigated. Moreover, by incorporating the conventional double emulsion method with a high throughput inertial microfluidics device, microspheres with a narrow size distribution have been obtained.
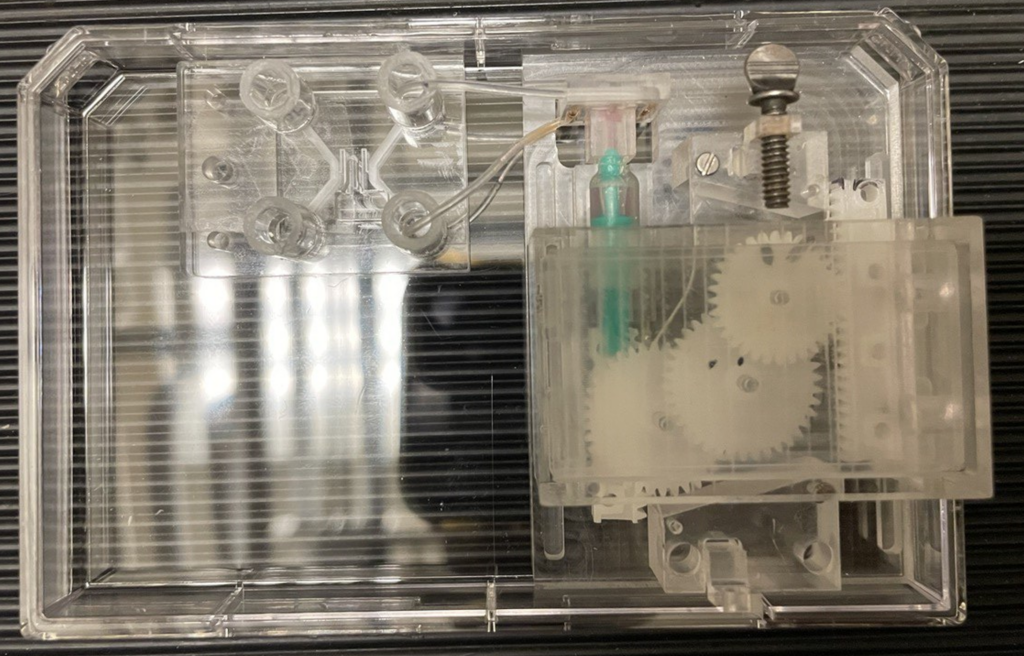
Fabrication of a mini rocker-based pump for organ-on-a-chip platforms
Most Organ-On-a-Chip (OOC) studies use commercial pumps, such as syringe and peristaltic pumps, which are large and heavy. These pumps have not been designed for OOC devices. They involve lengthy tubing, increasing the risk of bubble generation and consequent cell death. Furthermore, routine microscope imaging becomes cumbersome due to the need to disconnect the device from the pump. Addressing these limitations, the mini rocker-based pump was developed with two primary objectives: 1) to facilitate imaging and 2) to generate physiologic oscillatory flow in a short loop with minimal footprint. First, because of the small footprint of the planned pump design, it can be carried with the OOC device in a plate for microscope imaging and returned to the incubator without needing to connect and disconnect the tubes. Second, the pump can generate rectangular oscillatory flow with adjustable amplitude and frequency in a short loop, decreasing the chance of bubble formation.
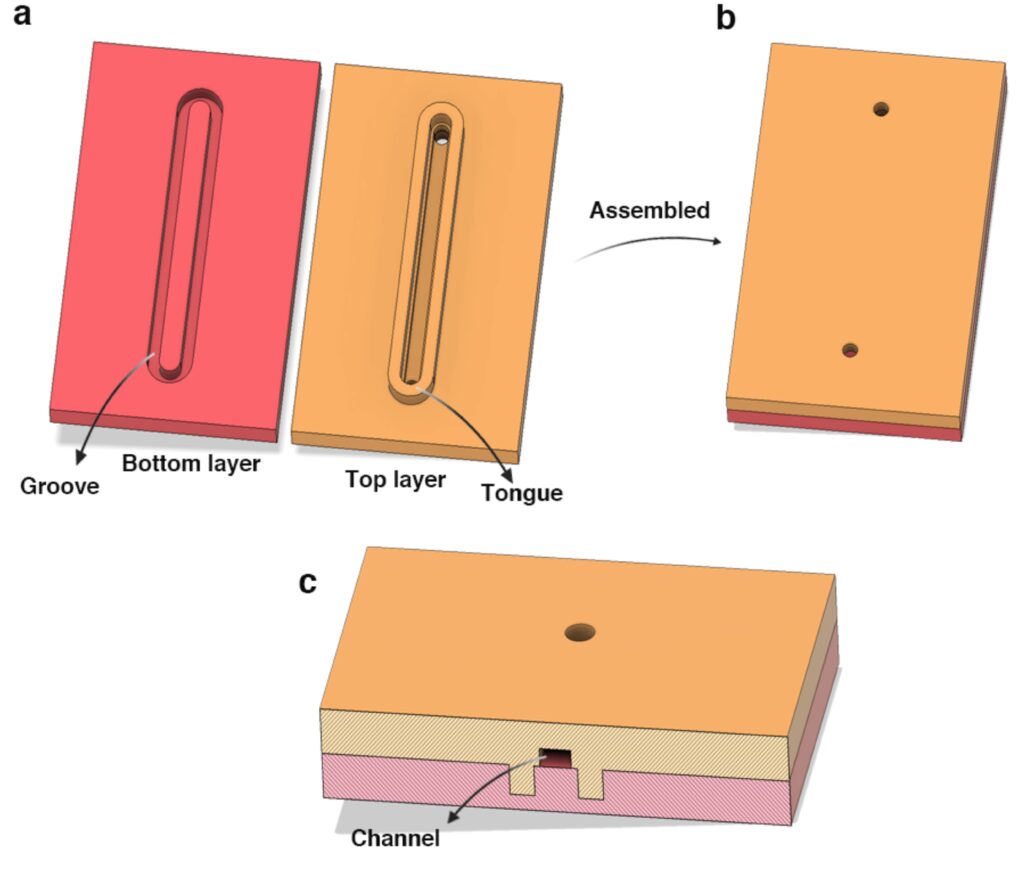
Developing a method for creating openable microfluidic devices
Organ-on-a-chip (OOC) devices can replicate in vivo conditions, but they often pose challenges for downstream analyses, e.g., SEM, AFM, and histological studies. They also lack real-time control over cell spacing. To address these limitations, in this project I developed a technique allows the creation of openable microfluidic devices without requiring any flexible layer (e.g. O-ring), or any adhesive liquid. Instead of using the common permanent bonding approach, a tongue and groove joint structure, similar to that found in Lego toys, is utilized to interlock two plastic PMMA layers. A simple example of a microfluidic device fabricated using this method is illustrated here. The top layer contains a microfluidic design, which itself contains a straight channel with inlet and outlet holes. An extruded tongue structure is present on the periphery of the design, while the bottom layer contains a groove with similar dimensions to the tongue (Figure a). Figure b shows the closed microfluidic device which is filled with red dye.
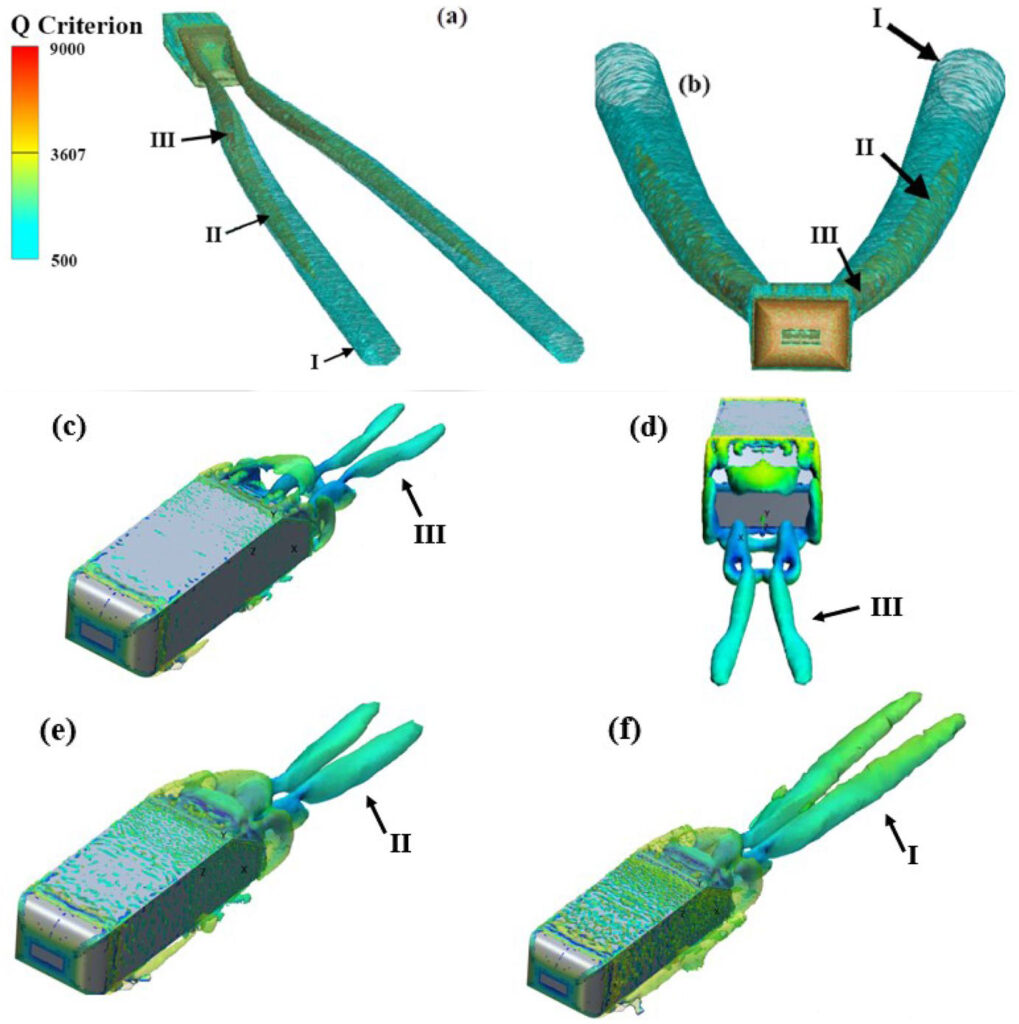
Numerical‑Experimental Geometric Optimization of the Ahmed Body and Analyzing Boundary Layer Profles
The trade-off between the fuel consumption and drag coefficient makes the investigations of drag reduction of utmost importance. In this paper, the rear-end shape optimization of Ahmed body is performed. Before changing the geometry, to identify the suitable simulation method and validate it, the standard Ahmed body is simulated using k − ω shear stress transport (SST) and k-epsilon turbulence models. The slant angle, rear box angle, and rear box length as variables were optimized simultaneously. Optimizations conducted by genetic algorithm (GA) and particle swarm optimization (PSO) methods indicate a 26.3% decrease in the drag coefficient. To ensure the validity of the results, a numerical-experimental study is conducted on the optimized model. Thereafter, the velocity profiles and flow structure in the boundary layers of the original geometry were compared to those of the optimized geometry at different sections. The results indicate that there are points where the velocity profile in the boundary layer can exceed the free stream velocity and return to it again, an overlooked observation in the previous studies. In addition to the streamlines, to better understand the formation of three-dimensional vortexes, the Q-criterion factor is computed and illustrated.
Videos
Using the CFD-Deep learning model for numerical simulation of red blood cells (RBCs) and circulating tumor cells (CTCs) separation from a patient’s blood sample in a spiral microfluidic device. Red trajectories represent CTCs, while blue trajectories indicate RBCs.
Assembling a Lego-inspired openable microfluidic device with a straight channel, featuring an inlet and outlet, each with its own reservoir.